A page from the "Causes of Color" exhibit...
Blue frogs and white lions (biological pigments)

The plant and animal kingdoms abound with bright colors, from the lush green of photosynthesizing plants to the bold black and orange stripes of tigers. Color plays a multitude of roles in the natural world, used to entice, to camouflage, or to warn other creatures. Colors signal harvest time, breeding conditions, and the change of seasons, from the first greens of spring to the brilliant reds and browns of the fall.
Pigments
Pigments are chemical compounds responsible for color in a range of living substances and in the inorganic world. Pigments absorb some of the light they receive, and so reflect only certain wavelengths of visible light. This makes them appear "colorful.” Cave paintings by early man show the early use of pigments, in a limited range from straw color to reddish brown and black. These colors occurred naturally in charcoals, and in mineral oxides such as chalk and ochre. The WebExhibit on Pigments has more information on these early painting palettes. Many early artists used natural pigments, but nowadays they have been replaced by cheaper and less toxic synthetic pigments.
This vibrant painting, The Milkmaid by Johannes Vermeer (c. 1658), shows his use of expensive pigments, including Indian Yellow, lapis lazuli, and Carmine. The only way to achieve a deep rich blue was by using the semi-precious stone lapis lazuli to produce a pigment known as ultramarine. Because the best sources of lapis lazuli were remote, the stone was expensive, and was later replaced by azurite and smalt (minerals) and indigo (biological). |
Historically, artists such as Titian used the pigment Vermilion to create the reds in the great fresco of Assunta, completed c. 1518. Vermilion is a mercury compound and has been replaced by convenient mixtures of synthetic, inorganic pigments. |
Some pigments have been categorized on this site in terms of the mechanism that causes a particular wavelength or group of wavelengths to be reflected rather than absorbed.
Ultramarine or lazurite (found in the gemstone lapis lazuli) is colored by the charge transfer process. The mechanism described by ligand theory causes the color of the pigment chrome green. Cadmium sulphide owes its distinctive color to the mechanism described by band gap theory. Lead compounds used to find widespread applications in white and gray paints, but are now rarely used because of their toxicity.
Pigments color paint, ink, plastic, fabrics, cosmetics, food, and a wide range of products we use and see all about us.
Biological pigments
Biological pigments are pigments produced by living organisms. They can be found in many plants, including flowers, and even in our skin. Bacteria are colored by pigments. All biological pigments selectively absorb certain wavelengths of light while reflecting others.
Color arises from the way the pigments react with light.
Biological pigments in plants
Plant pigments exist in a wide variety of forms, some with highly complex and large structures. Over 600 naturally occurring carotenoid structures have been identified, as well as over 7,000 flavonoids, including over 500 anthocyanins. This is discussed in more detail on the Flowers section. Biological pigments such as chlorophyll are colored organic molecules which owe their color to the presence of unsaturated bonds (e.g. C=C-C=C).
Biological pigments in animals
Melanin is the main pigment found in mammals. It is responsible for the color of hair and fur. There are different types of melanin (eumelanin and pheomelanin), and they produce a huge color range, from black to sandy to red.
A lion’s coloring is produced by melanin.
Tawny and brown shades of fur on this mandrill contrast with blue and red markings in areas devoid of fur.
Other ways that organisms create color
Fireflies and bacteria emit light in the form of bioluminescence. Luciferins are a class of light-emitting biological substance found in these organisms.
Although animals do manufacture their own melanin, they can’t make many other pigments. Plants, however, can produce a range of pigments, so many animals are colored by what they eat.
The eye-catching colors of many birds aren’t produced by the birds themselves, but by what they eat. Research into the varied plumage of a House Finch has shown that its colors are related to the bird’s diet and the pigments it eats. The carotenoid pigments of the tasty berries that the red Cardinal enjoys in the summer are laid down in the feather follicles. In the same way, yellow, red, and orange pigments can be absorbed from an array of seeds. The wide variety of summer fruits and seeds that form the bird’s diet as it prepares for the lean winter months also provides the pigments in its vibrant plumage. Another factor is the ability of the bird to metabolize carotenoid pigments to create plumage pigmentation of a different color than the ingested pigment. So, one type of seed, eaten by a House Finch, might make it appear yellow, while the same seed eaten by a Cardinal might make it appear pink, as the Cardinal converts the pigment metabolically to a red pigment. If you kept a colorful wild bird like a Cardinal or House Finch in captivity and fed it just one type of seed, its feathers would become progressively duller with each molt. |
lamingos eat planktonic animals such as brine shrimp. Both the flamingo and the shrimp are unable to make their own carotenoids. Microscopic algae manufacture red and yellow pigments, and form the primary diet of the tiny shrimp. When the flamingo dines on shrimp, the carotenoids move another step through the food chain to produce the vivid pink and oranges seen in the feathers. Most zoos supplement the diet of their flamingos with plant pigment extracts: gray flamingos would lack the visual appeal of the vivid colors we expect from these birds. Similarly, farmed salmon are fed a supplement to make them a more appetizing pink.
Invertebrates, such as insects or mollusks, often display green colors because of porphyrin pigments sometimes absorbed through their diet.
Unlike plants, most animals are unable to make green and blue pigments. Most of their green and blue colors are created through structural effects. A bluebird manufactures melanin and would look almost black, but tiny air sacs in the feathers scatter light and make it appear blue, in a similar way to the sky, which appears blue as gas molecules in the atmosphere scatter light. Peacocks are colored through a combination of pigments, and the way light interferes when reflected off the feathers to create iridescence. Examples of colors arising from iridescent and diffractive structures can be found in peacock feathers, [15B.html|]pearls, and mother of pearl. Another brilliant example of structural color in the animal kingdom is the brilliant blue of the Morpho butterfly. The color of their wings is the result of their microstructure, although many butterflies have cells that contain pigment as well. Some beetles with a metallic green sheen show similarly vibrant colors.
Structural color is the result of selective reflection or iridescence, usually because of multilayer structures. Pigment color differs from structural color in that it is the same for all viewing angles. Some colors are a combination of pigment, structural color, and diet. Most green colors in fish, reptiles, amphibians, and birds are created by a reflection of blue light coming through an over-layer of yellow pigment.
Blue Poison Arrow Frog from South America. Amphibians’ and reptiles’ skin contains three kinds of highly branched color cells called chromatophores. The chromatophores occur in three discrete layers. The top layer is generally made of xanthophores bearing yellow pigments; the middle layer includes iridophores; and the bottom layer has melanophores with black or brown melanin. In the typical green frog, light penetrates to the iridophores, which act like tiny mirrors to reflect and scatter mostly blue light back into the xanthopores above them. The xanthopores contain yellow pigments, and act as yellow filters so the light escaping to the skin surface appears green to our eyes. If a frog lacks the yellow xanthophores, blue light scatters back and the frog appears bright blue. Iridophores do not synthesize pigments, but reflect and refract color. They contain platelets that produce a scattering effect. The real advantage to these stacks of pigment cells lies in their potential to create color changes. The animal can darken its color by moving the melanin pigment. By manipulating the three types of cells, a wide range of colors can be produced, usually extending from bright green to shades of brown and grey. Generally xanthophores contain pteridines (synthesized) and are yellow, but they can produce red pigments. Sometimes the top layer may contain erythrophores as well as xanthophores. Erythrophores contain carotenoids (absorbed through diet) which produce intermediate colors like orange, reddish-orange, and yellowish-orange. The distinction is not always made, as sometimes pteridine and carotenoids are found in the same cell.
White animals
White animals are often found in nature and sometimes the cause is albinism. Melanin is the primary pigment that determines the color of a mammal`s skin, fur, and eyes. Albinism occurs in mammals (including humans), fish, birds, reptiles, and amphibians. It is a hereditary condition; the principal gene which results in albinism prevents the body from making the usual amounts of the pigment melanin. An animal inherits either a single trait or set of traits that interrupt melanin production.
Animals with albinism are typically white or very pale. However, not all animals with albinism are pure white; some traits that control melanin allow forms of the pigment to appear in the fur of the animal.
White bottlenose dolphin (possibly from albinism) |
An apparently albinistic humpback whale that travels up and down the east coast of Australia. |
The many types of melanin are responsible for brown, black, gray, and some yellow colorations. In some animals, especially albinistic birds and reptiles, ruddy and yellow hues or other colors may be present on the entire body or in patches (as is common among pigeons), due to the presence of other pigments unaffected by albinism such as porphyrins, pteridines, and psittacins, as well as carotenoid pigments derived from the diet.
Albinism in amphibians and reptiles can result in patterned skin in white, yellow or red. The color pattern of a normal corn snake is a mixture of black, red and white. In a corn snake lacking melanin (amelanistic) a red and white skin results. This is because amphibians and reptiles possess three types of chromataphores (pigment cells): melanophores, xanthophores and iridophores, in a similar skin structure to that of frogs. Melanophores contain brown or black pigments (melanin). Xanthophores contain red and yellow pigments. Iridophores contain crystals, which diffract the light, resulting in iridescence or scattering. In albinism the melanin is gone, and so the black pigment is removed and the snake appears red and white. The other pigments can also be affected by genetic conditions, resulting in a range of color combinations. Amphibians and reptiles can display a wide variety of color defects stemming from inherited deficiencies, or defects in the chemical process of making pigments. |
White lions (such as the one in front) are apparently not albinistic, but variants caused by a recessive gene. They are rare in the wild, are found in South Africa, and are often bred in zoos. Because the gene responsible is recessive, both parents need to carry the gene for white fur. |
White peacocks and white lions are examples of animals that appear white, but do not have albinism. The color of white lions has been attributed to leucism. Leucism is sometimes mistaken for albinism, but leucism is a condition characterized by reduced pigmentation in animals. It affects all pigments, not just melanin, and animals with leucism have normal eye color, while animals with albinism tend to have red eyes.
Big cats may be white through albinism or leucism, but in a related variation they can be black because they have melanism, an unusually high proportion of melanin. All these conditions relate back to their genetic makeup. In black leopards and jaguars with melanism, close examination will show that the markings are still there, masked by the black coloration. Melanism is known to occur in other species, but is common in cats.
Use of pigments for camouflage or defense
Pigmentation is used by many animals for protection, by means of camouflage, mimicry, or warning coloration. Pigments such as melanins in the skin may serve to protect tissues from ultraviolet radiation. Pigments may also aid in sexual reproduction, by identifying species and gender of animals to potential mates, or signaling readiness to breed.
It is now believed that chameleons change color not so much to blend into the surroundings, but rather to signal, fend off rivals, or attract a mate. The skin of a chameleon is similar to that of a frog and contains chromophores. The inner layer consists of melanin (melanophores), the next layer (iridophores) diffracts or scatters the light, and the top layer contains pigments (such as red and yellow). The expansion and contraction of the color cells is controlled by the chameleon’s brain to cause a color change. The melanin (which is black or brown) in the inner layer can move closer to, or farther away from, the skin surface, allowing it to blend in with, blot out, or intensify color from other cells. Another interesting fact about a chameleon is that they have a different visual system than humans and apparently have a fourth cone that is ultraviolet sensitive. How animals see is discussed more in animal vision.
Praying mantises are very well camouflaged, both to avoid predators and to ensnare their victims. Various species have adapted not only to blend with the foliage, but also to mimic it, appearing as parts of the plants they inhabit, or even stones.
The skin of an octopus changes almost immediately as it moves over different colored areas of the ocean floor. Color changes are controlled by small, elastic, pigment-filled sacs, known as chromatophores, found in the outer layer of the skin. The sacs are filled with red, yellow, or brown pigment, with some species having as many as five colors. When the muscles attached to each chromatophore contract, they pull the pigment sac outward to reveal an area of a particular color. When the muscles relax, the chromatophores close back up. The ink clouds a cephalopod produces complement this line of defense.
What do squid ink and your skin have in common?
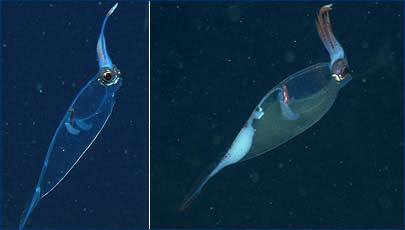
The left image shows a transparent squid, its ink invisible. On the right, the ink is clearly visible.
Squid, octopi, and cuttlefish are well-known for the jets of ink they squirt out in clouds at their attackers. This dark cloud distracts and confuses their potential predators. Cephalopods such as the octopus squirt ink composed primarily of concentrated melanin, produced in an ink sac. To add to the effect of this defense, the ink can contain among other things the chemical tyrosinase, a harmful compound that is thought to diminish sense of smell and cause irritation.
While most octopi have ink sacs, some, such as those that live the darkness of the deep sea, have reduced or absent ink sacs.
The melanin in octopus ink is red, but may appear brown or even black in high concentrations. Nocturnal and deep-sea cephalopods only produce red or brown ink, but in reduced light it appears black.