A page from the "Causes of Color" exhibit...
Why are hot things colored? (incandescence)

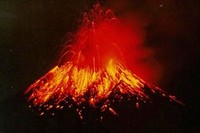
Volcanoes are a vivid example of incandescent molten rock
In the English language, we understand "white hot" to be hotter than "red hot," while "blue" is usually associated with degrees of coldness, as in "cool blue" or "icy blue." In terms of real temperature, "blue hot" is hotter than "red hot."
What is incandescence?
Incandescence is the emission of light by a solid that has been heated until it glows, or radiates light. When an iron bar is heated to a very high temperature, it initially glows red, and then as its temperature rises it glows white. Incandescence is heat made visible – the process of turning heat energy into light energy.
Our colloquial usage of "red hot," "white hot," and so on, is part of the color sequence black, red, orange, yellow, white, and bluish white, seen as an object is heated to successively higher temperatures. The light produced consists of photons emitted when atoms and molecules release part of their thermal vibration energy.
Incandescent light is produced when hot matter releases parts of its thermal vibration energy as photons. The Kelvin scale measures absolute temperature (a change of 1 K is equivalent to 1 °C), with 273 K being equivalent to water’s freezing point. At medium temperatures, say 1073 K (800 °C), the energy radiated by an object reaches a peak in the infrared, with a low intensity at the red end of the visible spectrum. As the temperature is raised, the peak moves toward and finally into the visible region. The temperature range experienced on earth, usually between 100 K and 2000 K, produces electromagnetic energy mostly in the infrared and visible light range, which gives us a convenient color temperature scale.
What is color temperature?
Light may be said to have a color temperature. Color temperature is a scale relating the color of light radiated by an object to its temperature. As color temperature rises, so the light emitted shifts towards bluer hues. In practice, the actual temperature is not the same as the color temperature, which is the reason correction factors are used.
The scale uses the colors of an abstract object called a black body radiator, which absorbs and then radiates all the energy that reaches it. This scale can be applied to a photographic lamp or even the sun, but it can also be applied to any source of light, using correction factors to allow for real surfaces not being perfect black body radiators.
For sources of light that do not rely on incandescence, such as fluorescent light, we use the correlated color temperature (CCT). These light sources will not produce light in the pattern of a black body emission spectrum. Instead, they are assigned a correlated color temperature, based on the match between human color perception of the light they produce and the closest black body radiator color temperature.
Here are the color temperatures of some common light sources:
|
When we talk about blue light being cool and red light being warm, we are referring to something very different from color temperature. We are using these colors to describe our perceptions or to convey moods. Counterintuitively, blue-hot is actually hotter than red-hot.
Black body radiation
Why use a black body radiator as a standard, when no such thing exists?
It turns out that black body radiation provides us with a set of very precise working equations that relate the temperature of an object to the light it emits. Working from the ideal and using Planck"s law, we can predict the energy distribution across the spectrum for a given temperature. The total emitted power is calculated using the Stefan-Boltzmann law. The wavelength of the peak emission, and hence the color that dominates for this temperature, is provided by Wien’s displacement law. Knowing the ideal case allows us to predict or calculate actual values by correcting for the imperfections of actual hot objects.
For increasing temperatures, the sequence of radiated colors is: black, red, orange, yellow-white, bluish-white.
Planck’s black body radiation curves for increasing temperatures. Planck’s work on deriving this equation led him to a breakthrough in understanding the quantum nature of matter. These curves also show the trend of shifting peak wavelengths for increasing temperature, as predicted by Wien.
Our definition of "white" is derived from emission from the 5800 K temperature near the surface of the sun. Its peak at near 550 nm (2.25 eV) is paralleled in the maximum sensitivity of our eyes in the same region. This is usually attributed to our evolution in the vicinity of our sun. No matter how high a temperature rises, blue-white is the hottest color we are able to perceive.
Incandescence from the sun
We can use the color of hot objects to estimate their temperatures from about 1000 K, as the peak wavelength moves into the visible spectrum. The tungsten filament light bulb, the most common manmade source of light on earth, glows at about 2854 K. The sun is a natural incandescent source whose surface, the photosphere, is about 5800 K.
The emission from the surface of the sun, with its average temperature around 5800 K, gives us our definition of white; its peak wavelength near 550 nm (2.25 eV) is mirrored in the maximum sensitivity of our eyes in the same region, reflecting our evolutionary progress while exposed to the light of the sun.
The sun’s energy is understood to come from nuclear fusion reactions at its core, with the center of the sun having an estimated temperature of around 15,000,000 K. As this energy travels outwards to the sun’s surface, the energy is transferred first by radiation (through a layer called the radiative layer), being absorbed and re-emitted at decreasing temperatures. Closer to the surface, through the convective layer, convection becomes the dominant mechanism for energy transfer as the sun’s plasma is less hot and dense here, and is unable to sustain heat transfer by radiation.
By the time it reaches the surface of the sun, the photosphere, it has reached the temperature of 5800 K that we perceive as visible white light.
In addition to heat and light, the sun also emits a low-density stream of charged particles (mostly electrons and protons) known as the solar wind, which travels throughout the solar system at about 450 km/sec. The solar wind and the much higher energy particles ejected by solar flares can have dramatic effects on the earth, ranging from power line surges and radio interference to the beautiful Aurora Borealis.
The bright white colors of fireworks are examples of incandescence. Metals, such as magnesium, are heated to white-hot temperatures during combustion. The other colors produced in pyrotechnical displays employ luminescence, rather than incandescence.
Other examples of incandescence and its uses
The color of incandescence is used to measure temperature in radiation pyrometers. Illumination sources, from the primitive candle through limelight, arc lamps, and the modern incandescent-filament lamps and flash bulbs, all use incandescence; usually the goal is to avoid color and create light as uniformly white as possible.
Metalworking relies heavily on incandescence to identify distinctive changes of temperature by color. Blacksmiths temper iron at red-hot temperatures, while jewelers need to know the color temperature of a particular metal to anneal it correctly, rendering it ready for working without under- or overheating it.
![]() A blacksmith removing a red-hot iron ingot from a forge. |
![]() The ideal annealing temperatures for silver (left) and gold produce the color temperatures shown: a dull pink for silver, and a red for gold. |