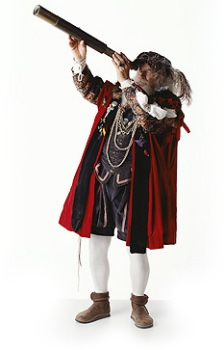
The principal astronomical cycles are the day (based on the rotation of the Earth on its axis), the year (based on the revolution of the Earth around the Sun), and the month (based on the revolution of the Moon around the Earth). The complexity of calendars arises because these cycles of revolution do not comprise an integral number of days, and because astronomical cycles are neither constant nor perfectly commensurable with each other.
What are different measures of the year?
The tropical year is defined as the mean interval between vernal equinoxes; it corresponds to the cycle of the seasons. Our calendar year is linked to the tropical year as measured between two March equinoxes, as originally established by Caesar and Sosigenes. The following expression, based on the orbital elements of Laskar (1986), is used for calculating the length of the tropical year:
365.2421896698 - 0.00000615359 T - 7.29E-10 T2 + 2.64E-10 T3 (days)
where T = (JD - 2451545.0) / 36525 and JD is the Julian day number. However, the interval from a particular vernal equinox to the next may vary from this mean by several minutes.
Another kind of year is called the sidereal year, which is the time it takes the earth to orbit the sun. In the year 2000, the length of the Tropical Year = 365.24219 days, and the length of the Sidereal Year = 365.2564.
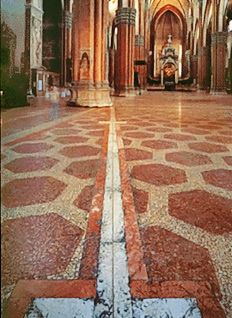
Meridan Line. S. Petronio, Bologna
In this sun calendar, a hole in the ceiling of the cathedral projects a shaft of sunlight onto this bronze strip on the pavement below, which is engraved with the days of the year and signs of the zodiac.
Astronomical Clock. Prague, Czech Republic
The Prague Astronomical Clock, which dates back to the 15th century, features a background illustrating the Earth and sky; an hourly clock; curved lines that represent 1/12 of the time between sunrise and sunset, and a circle with zodiac signs. A small star illustrates the vernal equinox. Sidereal time can also be read.
The synodic month, the mean interval between conjunctions of the Moon and Sun, corresponds to the cycle of lunar phases. The following expression for the synodic month is based on the lunar theory of Chapront-Touze’ and Chapront (1988):
29.5305888531 + 0.00000021621 T - 3.64E-10 T2 (days).
Again T = (JD - 2451545.0)/36525 and JD is the Julian day number. Any particular phase cycle may vary from the mean by up to seven hours.
In the preceding formulas, T is measured in Julian centuries of Terrestrial Dynamical Time (TDT), which is independent of the variable rotation of the Earth. Thus, the lengths of the tropical year and synodic month are here defined in days of 86400 seconds of International Atomic Time (TAI).
From these formulas we see that the cycles change slowly with time. Furthermore, the formulas should not be considered to be absolute facts; they are the best approximations possible today. Therefore, a calendar year of an integral number of days cannot be perfectly synchronized to the tropical year. Approximate synchronization of calendar months with the lunar phases requires a complex sequence of months of 29 and 30 days. For convenience it is common to speak of a lunar year of twelve synodic months, or 354.36707 days.
Three distinct types of calendars have resulted from this situation. A solar calendar, of which the Gregorian calendar in its civil usage is an example, is designed to maintain synchrony with the tropical year. To do so, days are intercalated (forming leap years) to increase the average length of the calendar year. A lunar calendar, such as the Islamic calendar, follows the lunar phase cycle without regard for the tropical year. Thus the months of the Islamic calendar systematically shift with respect to the months of the Gregorian calendar. The third type of calendar, the lunisolar calendar, has a sequence of months based on the lunar phase cycle; but every few years a whole month is intercalated to bring the calendar back in phase with the tropical year. The Hebrew and Chinese calendars are examples of this type of calendar.
Because calendars are created to serve societal needs, the question of a calendar’s accuracy is usually misleading or misguided. A calendar that is based on a fixed set of rules is accurate if the rules are consistently applied. For calendars that attempt to replicate astronomical cycles, one can ask how accurately the cycles are replicated. However, astronomical cycles are not absolutely constant, and they are not known exactly. In the long term, only a purely observational calendar maintains synchrony with astronomical phenomena. However, an observational calendar exhibits short-term uncertainty, because the natural phenomena are complex and the observations are subject to error.
What are Equinoxes and Solstices?
Equinoxes and solstices are frequently used as anchor points for calendars. For people in the northern hemisphere:- Winter solstice is the time in December when the sun reaches its southernmost latitude. At this time we have the shortest day. The date is near 21 December.
- Summer solstice is the time in June when the sun reaches its northernmost latitude. At this time we have the longest day. The date is near 21 June.
- Vernal equinox is the time in March when the sun passes the equator moving from the southern to the northern hemisphere. Day and night have approximately the same length. The date is near 20 March.
- Autumnal equinox is the time in September when the sun passes the equator moving from the northern to the southern hemisphere. Day and night have approximately the same length. The date is near 22 September.
For people in the southern hemisphere, winter solstice occurs in June, vernal equinox in September, etc.
The astronomical "tropical year" is frequently defined as the time between, say, two vernal equinoxes, but this is not actually true. Currently the time between two vernal equinoxes is slightly greater than the tropical year. The reason is that the earth’s position in its orbit at the time of solstices and equinoxes shifts slightly each year (taking approximately 21,000 years to move all the way around the orbit). This, combined with the fact that the earth’s orbit is not completely circular, causes the equinoxes and solstices to shift with respect to each other.
The astronomer’s mean tropical year is really a somewhat artificial average of the period between the time when the sun is in any given position in the sky with respect to the equinoxes and the next time the sun is in the same position.
Did the church study astronomy?
Yes, it did.
Although the Roman Catholic Church once waged a long and bitter war on science and astronomy (particularly condemning Galileo), in general, they were quite involved in astronomy. The church gave more financial and social support to the study of astronomy for over six centuries, from the recovery of ancient learning during the late Middle Ages into the Enlightenment, than any other, and probably, all other, institutions. The church was not necessarily seeking knowledge for knowledge’s sake, a traditional aim of pure science. Rather, like many patrons, it wanted something practical in return for its investments: mainly the improvement of the calendar so church officials could more accurately establish the date of Easter.
When to celebrate the feast of Christ’s resurrection had become a bureaucratic crisis in the church. Traditionally, Easter fell on the Sunday after the first full moon of spring. But by the 12th century, the usual ways to predict that date had gone awry. To set a date for Easter Sunday years in advance, and thus reinforce the church’s power and unity, popes and ecclesiastical officials had for centuries relied on astronomers, who pondered over old manuscripts and devised instruments that set them at the forefront of the scientific revolution.
In its scientific zeal, the church adapted cathedrals across Europe, and a tower at the Vatican itself, so their darkened vaults could serve as solar observatories. Beams of sunlight that fell past religious art and marble columns not only inspired the faithful but provided astronomers with information about the Sun, the Earth and their celestial relationship. Among other things, solar images projected on cathedral floors disclosed the passage of dark spots across the Sun’s face, a blemish in the heavens, which theologians once thought to be without flaw. Over the centuries, observatories were built in cathedrals and churches throughout Europe, including those in Rome, Paris, Milan, Florence, Bologna, Palermo, Brussels and Antwerp.
Didn’t the church condemn Galileo?
Yes. The traditional view of the church’s hostility toward science grew out of its famous feud with Galileo, condemned to house arrest in 1632 for astronomical heresy.
Since antiquity, astronomers had put Earth at the center of planetary motions, a view the church had embraced. But Galileo, using the new telescope, became convinced that the planets in fact moved around the Sun, a view Nicholas Copernicus, a Polish astronomer, had championed.
- Read Nicholas Copernicus, On the Revolutions.
- Read Galileo Galilei, Dialogue Concerning the Two Chief World Systems.
The censure of Galileo, at age 70, hurt the image of the church for centuries. In 1992, 359 years later, Pope John Paul II finally acknowledged that the church had erred in condemning the scientific giant. Although some scholars claim that Rome’s handling of Galileo made Copernican astronomy a forbidden topic among faithful Catholics for two centuries, in fact, Rome’s support of astronomy was considerable. The church tended to regard all the systems of the mathematical astronomy as fictions. That interpretation gave Catholic writers scope to develop mathematical and observational astronomy almost as they pleased, despite the tough wording of the condemnation of Galileo.
How did the observatories work?
Typically, the building, dark inside, needed only a small hole in the roof to allow a beam of sunlight to strike the floor below, producing a clear image of the solar disk. In effect, the church had been turned into a pinhole camera, in which light passes through a small hole into a darkened interior, forming an image on the opposite side.
On each sunny day, the solar image would sweep across the church floor and, exactly at noon, cross a long metal rod that was the observatory’s most important and precise part. The noon crossings over the course of a year would reach the line’s extremities which usually marked the summer and winter solstices, when the Sun is farthest north and south of the Equator. The circuit, among other things, could be used to measure the year’s duration with great precision.
The path on the floor was known as a meridian line, like the north-south meridians of geographers. The rod, in keeping with its setting and duties, was often surrounded by rich tile inlays and zodiacal motifs. The instruments lost much of their astronomical value around the middle of the 18th century as telescopes began to exceed them in power. But the observatories still played a significant role because the solar timepieces were often used to correct errors in mechanical clocks and even to set time for railroads.
One of the observatories also impressed Charles Dickens, who in his book Pictures from Italy wrote that he found little to like in Bologna except "the Church of San Petronio, where the sunbeams mark the time among the kneeling people." Today, the surviving cathedral solar instruments are lovely anachronisms that baffle most visitors, who are usually unaware of their original use or historical importance. In the book, The Sun in the Church, author Dr. Heilbron, describes his astonishment with seeing the old instruments in Bologna, Italy, at the Basilica of San Petronio. "The church itself was beautiful, somber," Dr. Heilbron recalled. "When the sun crawled across that floor, there was nothing else. That’s what you had to look at. It was intense."
In the great Basilica of San Petronio, a solar observatory was erected in 1576 by Egnatio Danti, a mathematician and Dominican friar who worked for Cosimo I dei Medici, the Grand Duke of Tuscany, and who advised Pope Gregory on calendar reform. The church observatory produced data long before the telescope existed. By 1582, the Gregorian calendar had been established, creating the modern year of 365 days and an occasional leap year of 366 days. Danti was rewarded with a commission to build a solar observatory in the Vatican itself within the Torre dei Venti, or Tower of the Winds. The golden age of the cathedral observatories came later, between 1650 and 1750, and helped to disprove the astronomical dogma that the church had defended with such militancy in the case of Galileo.
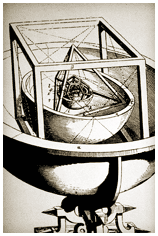
Kepler’s Model of the Universe
Another model of the heavens is that we’ve seen before is Kepler’s nested Platonic solids, and another is the dome. In The Dome of Heaven, Karl Lehmann, who writes, "One of the most fundamental artistic expressions of Christian thought and emotion is the vision of heaven depicted in painting or mosaic on domes..."
How did Cassini prove Kepler was right?
Among the best known of the rebel observers was Giovanni Cassini, an Italian astronomer who gained fame for discovering moons of Saturn and the gaps in its rings that still bear his name. Around 1655, Cassini persuaded the builders of the Basilica of San Petronio that they should include a major upgrade of Danti’s old meridian line, making it larger and far more accurate, its entry hole for daylight moved up to be some 90 feet high, atop a lofty vault. "Most illustrious nobles of Bologna," Cassini boasted in a flier drawn up for the new observatory, "the kingdom of astronomy is now yours." The exaggeration turned out to have some merit as Cassini used the observatory to investigate the "orbit" of the Sun, quietly suggesting that it actually stood still while the Earth moved. Cassini decided to use his observations to try to confirm the theories of Johannes Kepler, the German astronomer who had proposed in 1609 that the planets moved in elliptical orbits not the circles that Copernicus had envisioned.
If true, that meant the Earth over the course of a year would pull slightly closer and farther away from the Sun. At least in theory, Cassini’s observatory could test Kepler’s idea, since the Sun’s projected disk on the cathedral floor would shrink slightly as the distance grew and would expand as the gap lessened. Such an experiment could also address whether there was any merit to the ancient system of Ptolemy, some interpretations of which had the Earth moving around the Sun in an eccentric circular orbit. Ptolemy’s Sun at its closest approach moved closer to the Earth than Kepler’s Sun did, in theory making the expected solar image larger and the correctness of the rival theories easy to distinguish.
For the experiment to succeed, Cassini could tolerate measurement errors no greater than 0.3 inches in the Sun’s projected face, which ranged from 5 to 33 inches wide, depending on the time of year. No telescope of the day could achieve that precision. The experiment was run around 1655, and after much trial and error, succeeded. Cassini and his Jesuit allies confirmed Kepler’s version of the Copernican theory.
Between 1655 and 1736, astronomers used the solar observatory at San Petronio to make 4,500 observations, aiding substantially the tide of scientific advance.